
Excretion and osmoregulation
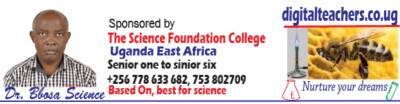

What is excretion?
Excretion is the elimination from the body of waste metabolic substances which if permitted to accumulate would poison the cells.
What is osmoregulation?
Osmoregulation is the process of maintaining salt and water balance (osmotic balance) across membranes within the body. Or Osmoregulation is the maintenance of the correct concentration of the body fluids at a steady state.
Waste metabolic products in animals
These waste metabolic substance include
- carbon dioxide,
- bile pigments,
- nitrogenous waste and
- toxic substances such as drugs, though are not products of metabolism.
The significance of excretion and Osmoregulation:
Functions of excretion and osmoregulation are:
- The removal of metabolic waste substances that would otherwise be poisonous to the body.
- Regulate the ionic content of the body fluids such as sodium, potassium, chloride and so on to ensure efficient metabolic and physiological processes.
- Regulate the water content of the body fluids
- Regulate the pH of the body fluids
Nitrogenous excretory products
Nitrogenous waste products are produced by catabolism of proteins and nucleic acids. The immediate nitrogenous waste product of the deamination of proteins is ammonia. This may be excreted immediately or converted into the major nitrogenous compound, urea, trimethylnitrogen oxide and uric. The structures of these wastes are:
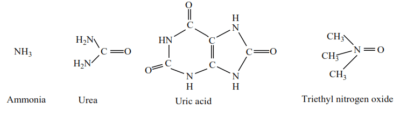
The exact nature of the excretory product is determined by
(i) the metabolic capability of the organism (i.e., which enzymes are presents);
(ii) the availability of water to the organism (i.e.; its habitat),
(iii) and the extent to which water loss is controlled by the organism.
Ammonia
It is an extremely soluble molecule and very poisonous is excreted with a lot of water. it’s thus, the main nitrogenous excretory products of aquatic invertebrate and all freshwater organisms since these organisms do not need to conserve water. Examples of organisms that excrete mainly ammonia are protozoan e.g. amoeba, cnidarian, Platyhelminthes, larval amphibian, aquatic crustacean, and echinoderm.
Urea
Urea is formed in the liver of vertebrates by the interaction of ammonia, produced by deamination, and carbon dioxide, produced by respiration in a cyclical reaction known as the urea or ornithine cycle. It is less soluble and less toxic than ammonia and is the main nitrogenous excretory product in cartilaginous fish, certain bony fish; adult amphibian and mammal.
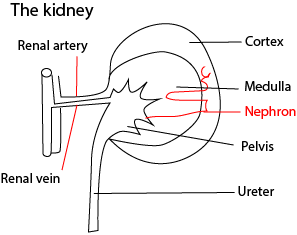
Uric acid
Uric acid and its salts are ideal excretory product for terrestrial organism and a pre-requisite for organism producing a shelled egg since they combine a high nitrogen content with low toxicity and low solubility.. They are stored in cells, tissue and organs without producing any toxic or adverse osmoregulatory effect, and they require a minimal amount of water for their excretion. It is the main excretory products in birds and insects where it is removed as solid pellets.
The disadvantage of excreting uric acid is that it requires more energy for production than urea than ammonia.
Trimethylamine oxide
This is produced by the addition of methyl groups to ammonia, formed by deamination, and the subsequent oxidation of inter-mediate molecules. Trimethylamine oxide gives fish its characteristic smell.
Excretory organs in animals
These include
- the skin excretes water, urea and salts
- lungs excrete carbon dioxide
- The liver removes old red blood cells and forms bile pigments
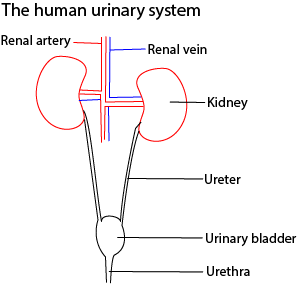
The mammalian urinary system is composed of two Kidneys, that control the composition of the body fluids by selecting, and removing unwanted substance from blood; ureter, convey urine from the kidneys to the urinary bladder; urinary bladder, stores urine temporarily before it is passed out of the body the urethra.
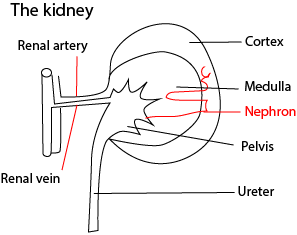
The kidney consists of two main regions: an outer cortex and inner medulla. Each kidney contains between one and two million microscopic structures called nephrons. The nephron is the basic unit of the kidney in which the kidneys regulatory functions such as producing urine are carried out.
Structure of a nephron
The main parts of the nephron are shown in the figure above. Part of the nephron lies in the cortex, and part of it in the medulla.
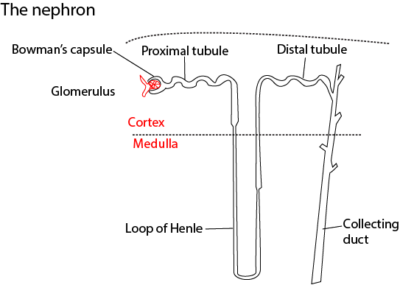
The nephron consists of a cup-shaped Bowman’s capsule which is like a hollow rubber ball that has been pressed on one side. The invagination contains a dense network of capillaries called a glomerulus. The inside of the capsule, the capsule space, is separated from the lumen of the capillaries’ by only two layers of cells: the epithelium of the capsule and the endothelium of the capillaries. These two layers of cells are highly specialized.
Leading from the Bowman’s capsule is a tubule, about 60µm in outer diameter, whose lumen is continuous with the kidney capsule space. The first part of the tubule is located in the cortex of the kidney and is coiled: It is called the proximal convoluted tubule.
The proximal convoluted tubule leads to a U-shaped loop of Henle, named after its discoverer. F. G. S. Henle, a German microscopic specialist in 19th century. It consists of a straight descending limb which enters down into the medulla, where it does a hairpin bend and returns to the cortex as the ascending limb. The ascending limb of the loop of Henle leads on to the final part of the nephron, the distal convoluted tubule. This, as the name applies, is coiled and it opens into a collecting duct. Several nephrons share the same collecting duct.
The collecting ducts converge at the pelvis of the kidney, emptying their contents into the ureter which conveys them to the bladder as urine. The tubules and collecting ducts are lined with a single layer of epithelial cells mainly of the cuboidal type.
Each nephron has its own blood supply. Blood from the renal artery is carried to the glomerulus by the afferent arteriole and leaves it by then efferent arteriole. The latter splits into a capillary network which envelops the tubules of that particular nephron. Blood from the capillaries drains into the renal vein. Blood flows first to the glomerulus and then capillaries surrounding the tubules before leaving the kidney.
The kidney processes for urine formation
- Ultra-filtration: All small molecules. such as water, glucose, and urea, are filtered out of blood plasma in the glomerulus and produce a filtrate in Bowman’s capsule which passes into the tubule of the nephron. The high filtration pressure results from the fact that in each Bowman’s capsule the afferent arteriole has a larger diameter than efferent arteriole. As a result of this pressure, substances are forced out of blood into capsule space. Big molecules like proteins and blood cells are retained in blood by the basement membrane of the glomerular capillaries and do not appear in the filtrate.
- Selective re-absorption: All substances useful to the body and required to maintain the water and salt composition of the body fluid at a steady state are removed from the filtrate and re-absorbed into the blood capillaries,
- Glucose, water, salts, amino acids are absorbed in the proximal convoluted tubule.
- Water is mainly absorbed in the loop of Henle
- In the distal convoluted tubules substances like water and salts are absorbed according to need as fine adjustment of body fluids.
- Collecting duct mainly absorb water
- Secretion: Substances such as ammonia, uric acid and urea not required by the body that were not filtered are secreted into the filtrate, by cells of the nephron before it leaves the kidney as urine, for example K+, H+ and NH4+ in the distal convoluted tubes. Potassium ions are secreted and re-absorbed: their concentration in the blood depends on the balance between the two opposing processes.
Basic mechanism of absorption and secretion in the tubules.
- Active transport: e.g. glucose is absorbed from proximal tubules by active transport.
- Differential permeability: Various regions of the nephron are selectively permeable to ions, water and urea, for example the distal tubules are relatively impermeable compared to proximal tubule; the permeability of the collecting duct can be modified by hormones.
- Concentration gradients: A concentration gradient varying from 300mosmkg-1 of water in the cortex to 1200 mOsmkg-1 of water at the papillae, is maintained within the interstitial region of the medulla in human and results in passive absorption of water.
- Passive diffusion and osmosis: Sodium and chlorine ions and urea molecules will diffuse either into or out of the filtrate, according to the concentration gradients, wherever will pass out of the filtrate into a concentrated fluid in the interstitial region of the kidney wherever the nephron is permeable to water.
- Hormonal control: The regulation of water balance in the body and salt excretion is achieved by the effect of hormones acting on the distal tubule and collecting duct, such as anti-diuretic hormone, aldosterone, etc.
The role of the loop of Henle in production of concentrated urine.
The loop of Henle concentrates urine by a counter-current flow mechanism. Here urine flows down the descending loop and then exactly in opposite directions in ascending loop.
- The descending loop of Henle is fully permeable to water and sodium chloride here water is absorbed by osmosis.
- The ascending loop of Henle is impermeable to water thus less water is absorbed from the filtrate. However, sodium and chloride ions are removed by active transport and returned to the medulla. This coupled with sluggish medullary blood, which reduces the rate of removal of salts from the medulla, maintains a high salt concentration in the medulla enabling maximum absorption water from the descending loop of Henle and the collecting duct.
In summary of events in Loop of Henle and collecting duct;
- Down the descending loop of Henle, the concentration of urine increases due to passive reabsorption of water.
- In ascending loop of Henle, the concentration decreases due to the removal of sodium and chloride ions by active transport.
- Down the collecting tubule concentration increases due to the passive absorption of water.
- An organism living in dry areas has big medulla and long loops of Henle to maximize water reabsorption and conservation.
- Absorption of water in distal convoluted is regulated by hormones such as antidiuretic hormone and aldosterone.
Kidney as osmoregulator
Drinking, particularly if excessive, results in the osmotic pressure of the blood to fall below normal value. The osmoreceptors are less stimulated leading to less ADH being produced. Less water is thus reabsorbed from the tubule leading to copious dilute urine (diuresis).
The inability of a person to produce insufficient amounts of ADH causes diuresis; the disease condition is called diabetes insipidus.
Note that the production of hypertonic/hypotonic urine is determined by the distal convoluted tubules by the presence or absence of ADH. Presence of ADH leads to reabsorption of water from the filtrate leading to hypertonic urine.
Control of sodium levels in the blood
Another hormone called aldosterone responsible for maintaining a more or less constant sodium level in the plasma has a secondary effect on water reabsorption.
Any loss of sodium which causes a decrease in blood volume causes a group of secretory cells called juxtaglomerular complex between the afferent arteriole and the distal convoluted tubule to release enzyme renin.
- Renin causes a plasma globulin produced by the liver to form hormone angiotensin.
- Angiotensin stimulates the release of aldosterone from the adrenal cortex.
- Aldosterone stimulates active uptake of sodium ions and consequently water from glomerular filtrate restoring the volume of blood.
pH control
Proteins, hydrogen carbonate and phosphate buffers in the blood prevent excess hydrogen ions (H+), produced by metabolic activities from decreasing the pH of the blood. Carbon dioxide released into the blood during respiration is regulated by this system and prevented from causing changes in blood pH prior to excretion in the lungs.
However, changes in pH of blood is counteracted by the distal convoluted tubules. In the distal convoluted tubules, carbon dioxide reacts with water to form hydrogen ions and hydrogen carbonate.
When the blood pH decrease, the distal convoluted tubules secretes H+ into tubules and retains HCO3–. H+ reacts with ammonia for form ammonium ions (NH4+) that is excreted in the urine.
When the pH of blood rises, the tubules secrete HCO3– ions and retain H+ lowering pH.
Excretion and osmoregulation in other organisms.
- Marine invertebrates:
The internal osmotic pressure (Opi) of some of these organisms is equal to external osmotic pressure (Ope). This implies that their body fluids are isotonic to seawater, these organisms do not need an osmoregulatory mechanism so long as they remain in the open seas.
However, some species of crab are capable of osmoregulation enabling them to move to estuarine water and freshwater. There are therefore three crabs to consider;
- Spider crabs (Maia) are confined in seawater because they lack any form of osmoregulation mechanisms.
- Shore crab (Carinas) is capable of some form of osmoregulation when it moves from sea to estuarine, where it maintains fairly high Opi above Ope but its osmoregulation mechanisms fail in freshwater. Hence it lives in seawater and estuarine only.
- Mitten crab. This type can osmoregulate in seawater and freshwater maintaining its Opi. It can, therefore, live in seawater, estuarine and freshwater.
A graph showing variation of Opi of different species of crabs with Ope of the medium
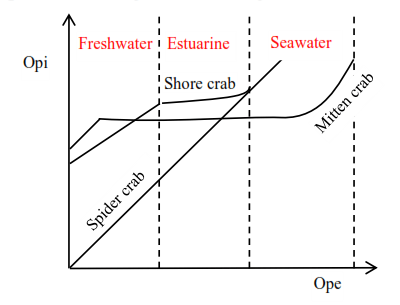
Freshwater animal
The problem facing a freshwater animal is that opi is greater than ope. The danger here is the dilution of the tissue fluid resulting from the osmotic influx of water across the exposed partially permeable surfaces of the body.
There are two possible solutions to this problem:
a. Water might be eliminated as fast as it enters the body fluid by means of the kidney or some equivalent device, salt being re-absorbed from the water before it leaves the body as done in an amoeba.
Osmoregulation in Amoeba
The internal osmotic pressure (OPi) of amoeba is always higher than the external osmotic pressure (OPe) , Osmotic influx of water can cause their membranes to burst.
- Excess water collects in the contractile vacuole;
- The salts from water are actively removed and returned to the cell.
- When the contractile vacuole is full, it migrates and fuse with the cell membrane and burst to release water to the surrounding.
b. Salts might be actively taken up from the external medium, thereby counteracting the diluting effect of the water inflow. For instance, the freshwater bony fish such as carp, trout, and stickleback are liable to an osmotic influx of water across the gills and the lining of the mouth cavity and pharynx. The freshwater bony fish must get rid of water and save salts.
This is achieved in three main ways:
- The rate filtration in the kidney (glomerular filtration rate) is very high, to allow rapid excretion of water. This high filtration rate is achieved by the glomeruli being exceptionally large and numerous.
- As the renal fluid flows along the kidney tubules, salts, (i.e.; sodium and chloride ions) are extensively re-absorbed back into the bloodstream with the result that urine is markedly hypotonic to blood.
- Chloride secretory cells: In the gills take up sodium and chloride ions from water by active transport into the bloodstream. The result is that chloride ions are about 800 times as concentrated in animal blood as it is in the surrounding freshwater.
Thus, to summarise, fresh waterway fish solve their osmotic problem by combining the expulsion of water with the active uptake of salts.
Marine vertebrate:
The hypertonic marine water exposes the marine vertebrate to chances of loss of water by osmosis. However, marine bony fish such as mackerel and cod are able to overcome this by three processes.
- The glomerular filtration rate in the kidney is relatively low. The glomeruli are small and few compared to those of the freshwater fish.
- Salts are actively extruded by chlorine secretory cells in the gills
- Nitrogenous waste is excreted in the form of urea and trimethylamine oxide) that require relatively little water for its elimination
- Marine cartilaginous fish (elasmobranchs and shark) such as dogfish and sharks have solved their osmoregulatory problems by retaining urea to increase the osmotic potentials of their body fluids to slightly above that of seawater. As a result, the little water that flows in readily expelled by the kidneys
- They have membranes that are impermeable to urea.
Adaptation of desert frogs to desert condition
- Desert frogs burrow underground during the dry months, in order to escape the searing sun. They can stay underground for many months while they wait for the next rains to fall.
- Whilst underground, many species produce a type of cocoon with many layers of their shed skin that covers their entire body (except for the nostrils). This helps to reduce water loss.
- Most desert frogs only reproduce in response to a significant rainfall event. Females can then lay eggs in temporary pools. Some even lay their eggs in mud. These eggs then hatch when they are eventually flooded with water, and the tadpoles can swim straight into the water! Smart.
- One particular type of frog, the Northern Sandhill Frog, doesn’t even need water to hatch its eggs. These babies hop right over the tadpole stage, hatching out of their eggs as tiny, fully formed versions of their adult parents.
- Chiloleples, the desert frog flourishes in the desert because it is nocturnal that hides during day when temperatures are high and become active during night when temperatures are low
Terrestrial animals
Like marine bony fish, terrestrial animals are liable to lose water, but whereas in a fish this is caused by osmosis, in a terrestrial animal it is caused by evaporation from the permeable surfaces exposed to the atmosphere.
Means of reduction of excess water loss from terrestrial animals.
- They have a waterproof integument, e.g. insects.
- They have is low glomerular filtration rate.
- They produce a non-toxic nitrogenous waste; for example, amphibians and mammal excrete urea which, being less toxic than ammonia requires less water for its removal. Reptiles and insects excrete uric acid in a semi-solid form. In insects, the uric acid is produced by the Malpighian tubules a bunch of narrow tubes leading to the gut.

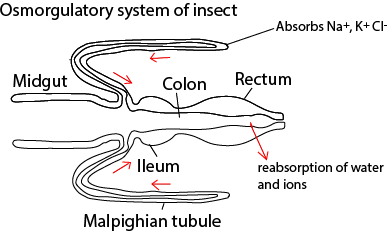
4. Reabsorption of water
In insects, water is mainly reabsorbed in the rectum while in mammals and birds, reabsorption occurs in the loop of Henle. High reabsorption in desert animals is due to the long loop of Henle while kangaroo rat which produces 4 times concentrated urine as that of humans has unusually high ADH.
5. Behavioral means
Many animals avoid or at least reduce, the problem of water loss by modifying or changing their habitats. For instance, kangaroo rat and earthworm burrow during hot days. Certain animals go into a state of dormancy during summer or dry season, a phenomenon called aestivation.
6. Use of metabolic water:
E.g. a desert animal such as kangaroo rat tends to metabolize fats rather than carbohydrate to obtain a high yield of water.
7. Tissues tolerant to dehydration
Many desert animals’ tissues such as the camel tissues are exceptionally tolerant to dehydration.
Water regulation in plants:
Plants can be classified into three groups according to how much water is available to them in their natural environment
.1. Hydrophytes: are plants which live partially or completely submerged in freshwater. e.g. water lilies, obviously, such plants have no difficulty getting enough water. Hydrophytes have the following adaptations to get rid of excess water
(i) Poorly developed root system to reduce water absorption.
(ii) Numerous stomata on the upper epidermis of the leaf to increase water loss.
(iii) Have thin epidermis on the leaves
(iv) Have poorly developed xylem to reduced water transported in plant
(v) Broadleaves
2. Mesophytes: grow in normal, well-watered soil. Most land plants in temperate regions belong to this category. Usually, the water which they lose by transpiration is readily replaced by uptake from the soil, so they require no special means of conserving water.
3. Xerophytes: live in dry places such as the hot desert where the water potentials in the soil and air are very low. These plants face the possibility of drastic dehydration and have ways of preventing this.
Adaption of xerophytes to drought
- Life cycle adaptation.
Most species can survive dry periods in the dormant stages e.g. desert ephemerals. Germination, growth, and flowering take place during a few weeks following a burst of rain after the seeds have been dispersed, the parent plant die and the seeds remain dormant in the dry soil until the next rains come.
Adaptation relating to transpiration and water uptake. Many species have adaptations that minimize transpiration and/or maximize water uptake.
These include:
- Extremely long vertical roots absorb water deep in the soil e.g. Acacia.
- Superficial roots have the advantage of absorbing water quickly before it has a chance of evaporation e.g. cacti.
- Some plants have got succulent leaves and/or stem to store water e.g. giant saguaro cactus of North America.
- The number of stomata in some plant e.g. in prickly pear has been reduced; in others the stomata are sunk down pits in which air tends to accumulate, thus reducing the rate of transpiration from the leaves. Some plants achieve the same thing by having a hairy epidermis which holds humid air against the leaf surface. These devices are accompanied in some plants by folding of leaves, an adaptation seen in marram grass, which thrives on dry coastal sand dunes.
- Cuticular transpiration in xerophytes is reduced by having small leaves with low surface area and by having a thick cuticle that is impermeable to water. In some plants, leaves have been modified into thorns.
- Some plants may suffer from water shortage in winter because of the freezing of soil which decreases water availability from the soil, causing physiological drought. One way of circumventing this problem is to shed the leaves by deciduous trees before winter sets in, thereby reducing the leaf surface area.
Crassulacean acid metabolism: a physiological adaptation
In Crassulacean, prevent excess loss of water by closing their stomata during day and then opening then at night. These plants have got the ability to take up carbon dioxide and fix it into malic acid at night when the stomata are open. When day break comes, the stomata close and carbon dioxide are released for photosynthesis.
Summary of challenges faced by vertebrates when they moved from water and evolutionary solutions
Challenges | Solution | |
1. | Obtaining gases | Development of lungs |
2 | Excessive evaporation | Impervious body coats e.g. shells, skin and cuticle and secretion of non-toxic wastes |
3 | Reproduction | Internal fertilization |
4 | Support and locomotion | Development of limbs |
5 | More predators | Many defence mechanisms |
Plants do not possess special excretory organs like animals because:
- Excretory products are gaseous and diffuse out through the stomata e.g. oxygen produced in photosynthesis and carbon dioxide in respiration.
- Waste products (oxygen, carbon dioxide, ammonia ) are reusable.
- plants are inactive and produce little waste products.
- waste products like resins are stored in the vacuole and lost with falling old leaves
Summary of ways by which plants excrete their metabolic wastes products.
- Gaseous waste (oxygen and carbon dioxide) by diffusion through the stomata and lenticel
- Stored solid and liquid waste by shedding leaves, peeling of bark and falling of fruits.
- By secreting waste in the form of gum and resins
Sample question and answers
Download samples questions and answers for O- and A -level from the links below
Perfectly pent subject material, regards for selective information “Life is God’s novel Let him write it” by Isaac Bashevis Singer
You have a gift for explaining things clearly. Stationary
Thanks for shedding light on this. Tech News
Top MBBS Colleges in Uttar Pradesh are known for their advanced infrastructure and quality education.
Win exciting rewards and enjoy seamless gaming on Raja Luck.
Stay updated on everything related to Raja Luck.