
Gaseous exchange in animals
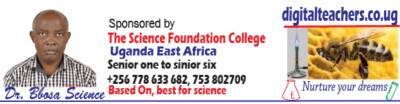
Gaseous exchange.
Whether aerobic or anaerobic respiration is occurring the constant passage to gases between an organism and their environment has to be maintained. Aerobes require oxygen for oxidation of foodstuffs to release energy whilst aerobes and most anaerobe must expel carbon dioxide, a waste product of respiration. Exchange of carbon dioxide and oxygen between the environment and the organism is termed gaseous exchanges, and the area where gaseous exchange actually takes place is called the respiratory surface.
Adaptations of a respiratory surface.
- It must be permeable so that gases can pass through.
- It must be thin because diffusion is only efficient over distances of 1mm or less.
- It should possess a large surface area so that sufficient amounts of gases are able to be exchanged according to the organisms need.
- It must be moist to ease the diffusion of gases across the respiratory surface.
In small animals, the surface to volume ratio is large enough for diffusion across the external surface to satisfy their respiratory need.
Bigger animals, particularly active ones have small the surface to volume ratio which is inadequate to supply enough oxygen to satisfy their metabolic needs. They have overcome this problem developing special respiratory surface which increases the efficiency of gaseous exchange. In addition, their blood contains respiratory pigment to increase oxygen-carrying capacity of the blood.
Specialized gaseous exchange surfaces
The specialised gaseous exchange surfaces provide large surface area by developing flaps, sac or tubes.
Examples
- Body surface is usually sufficient gaseous exchange surface for small animals such as protozoa, earthworm, flatworms with larges surface-volume ratio.
2. Aquatic organism such as lungworm and tadpole use external gill which are epidermal outgrowths from the surface of the body


3. Fish use internal gills which are enclosed within the body and protected from damage.


4. Air-breathing vertebrate have developed lung where air is brought close to blood to allow gaseous exchange


The biggest problem of gaseous exchange through by the lung is that energy is required for ventilation especially during inspiration and leads to water loss.
5. insects have developed a tracheal system penetrating and ramifying and delivering oxygen to tissue cell
6. The frog and other amphibians use the skin and buccal cavity in addition to lungs
Gaseous exchange in arthropods.

Gas exchange in insects takes place through the trachea, the air tubes, which terminally branch in tracheoles. Each tracheole delivers oxygen and removes carbon dioxide from each cell. Air enters and leaves the tracheal system through the spiracles.
Some insects can ventilate the tracheal system with body movements.
The gaseous exchange then occurs directly between the air in the tracheoles and the tissues and blood is not required to carry the gases. Tracheoles lack chitin moreover the degree of their branching may be adjusted according to metabolic needs of individual tissues.
In some insects such as grasshopper are ventilated by rhythmic movements of the thorax and abdomen and in all flying insects, ventilation is aided by muscular movement during flight.
Control of ventilation rate in insects
Insects control the rate at which oxygen is delivered to the tissues by
- Regulating the opening of the spiracles by the tinny muscles controlled by chemoreceptors. When the spiracles are open-air enter the tracheal system and are able to reach the cells
- The ends of the tracheoles are fluid-filled. At rest the tissues have a lower solute concentration than the fluid in tracheoles. As activity increases the muscles respire anaerobically and lactic acid accumulates. This raised the solute concentration of these cells above that of fluids in the tracheoles. Water therefore moves out of the tracheoles into the muscle cells by osmosis allowing more air to reach the cell. When the activity ceases the metabolites are oxidised, the solute concentration of the muscle is lowered and water re-enters the tracheoles reducing the amount of air reaching the cell.
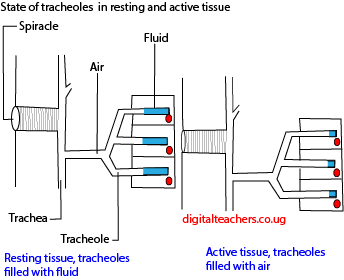
3. Ventilation is increased by muscular movement during flight.
Adaptations of insects to effective gaseous exchange
- It has got a tracheal system that ramifies the whole-body delivering oxygen to each cell.
- The extent of this ramification is adjusted to meet the tissues’ need.
- Insects have a control mechanism that supply the right amount of air to the cell as required from time to time.
- Insects are small and flattened to ease the diffusion of gases.
The disadvantage of the tracheal system
It limits the size of an insect because it relies entirely on diffusion for the gases to move from environment to the respiring cells.
It lacks effective ventilation system
Gaseous exchange in Bony fish
Gaseous exchange between fish and water occurs over the gills located in the opercular cavity which is enclosed by muscular flap, the operculum. Water is drawn into and pumped out of the pharynx by the movement of the operculum
Inspiration
Water is sucked through the mouth by an expansion of the buccal cavity and then into the opercular cavity by the outward movement of the operculum accompanied by contraction of the buccal cavity
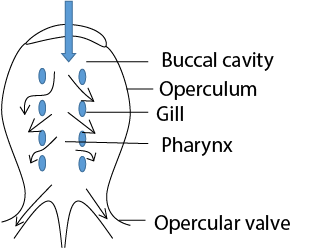
Expiration
Water is expelled through the opercular opening by inward movement of operculum together with continued contraction of buccal cavity. The mouth closes and operculum valve opens.
As water passes over the gills, gaseous exchange (oxygen into blood and carbon dioxide outside) occur between water and blood. The ventilation cycle maintains a continuous stream of water over the gills at all times. This ensures their efficiency.
This is a system developed by the fish in order to achieve maximum gaseous exchange. In this system blood and water flow in the opposite direction along any gill lamellae. This means that as blood flows across the respiratory surface, it approaches water which has more and more oxygen available for exchange.
The advantage of the counter-current flow system is that a stiff diffusion gradient is maintained throughout the gaseous exchange surface.
Graphical representation of the counter-current flow system

Parallel flow system
Here water and blood flow in the same direction at the same speed. The concentration gradient will be great at first, however, it will steadily decrease as blood flow together with blood long the distance of the gill lamellae.

Parallel flow is not therefore very efficient because blood leaves the gill less saturated than it would in the counter-current flow. It can, however, be improved when the speed of water is faster than the speed of blood.
Adaptations of the fish gill to gaseous exchange
- Gills are red- rich blood supply
- many tiny gill filaments increase surface area for gaseous exchange
- Gill lamellae are flat to help increase their surface area for oxygen exchange
- Water enters the mouth and comes out through operculum
- Water passes over the gills
- The epithelium covering gills is only one cell thick reducing diffusion gradient.
- Gill filaments are protected from damage by an operculum
Gaseous exchange in amphibians.
A frog is able to exchange gases in three different ways
- Through the skin by cutaneous respiration
- Via the epithelium, if the buccal cavity: buccal respiration and
- In the lungs: pulmonary respiration.
Gaseous exchange in man
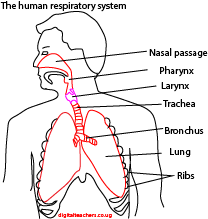
The human lungs and associated structure are shown in figure above. The lungs are situated in the thorax, the walls of which are formed by the ribs and intercostal muscles and the floor of the diaphragm. The lungs are surrounded by a very narrow pleural cavity lined by pleural membranes. The pleural cavity contains a thin layer of lubricating which allows the pleural membranes to slide easily over each other as the thorax expands and contracts during breathing.
Inspiration.
Air is drawn into the lungs via the trachea and bronchi.
- External intercostal muscles contract and rise the ribs upwards and outwards.
- The radial and circular muscles of the diaphragm contract and diaphragm flattens.
- There is an increase in the volume of the thoracic cavity and a decrease in pressure in the lungs.
- Air is drawn into the lungs to equalise the pressure to atmospheric pressure.
Expiration.
- This is a reverse of the inspiration process; air being expelled from the lungs.
- It is mainly a passive process resulting from elastic recoil of the tissues that have been stretched during inspiration.
- However, in forced breathing or when breathing tubes are blocked, expiration is aided by contraction of the internal intercostal muscles and abdominal muscles.
- Contraction of the latter raises the pressure in the abdominal cavity, forcing the diaphragm upwards.
Alveoli.
Alveoli (singular alveolus) are numerous hollow, lobed air sacs that form the gaseous exchange surface in the lungs.
They occur at the terminal end of the tracheal branching and come in close association with the extensive capillary system.
This makes them efficient apparatus for gaseous exchange.
Diagram of alveolus showing an intimate association between alveoli and blood capillaries.

Adaptations of the alveolus to efficient gaseous exchange
- They are very numerous that they offer a big surface area for gaseous exchange
- Alveolar epithelium is covered internally with a thin layer of fluids in which oxygen dissolves before diffusing into the blood which creates high diffusion gradient.
- Alveolar fluid contains surfactants that prevent alveolar from collapsing
- Alveolus is separated from blood capillaries by thin membrane that offers minimum resistance to diffusion of gases.
- The blood capillaries of the alveolus are smaller than the red blood cells such that when cell squeeze through the capillaries, they make intimate contact with capillary membranes that ease gaseous diffusion.
The ventilation cycle.
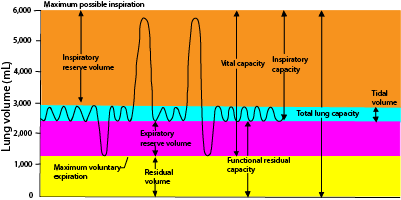
Pulmonary ventilation is accomplished by expansion and contraction of lungs.
Description of lung volumes
- tidal volume; is the volume of air breathes in and out at rest. It about 0.5L.
- Ventilation rate; is the volume of air breathed per minute. It can be obtained by multiplying the tidal volume with the number of air is breathed in and out per minutes
- Inspiration reserve volume; is the extra volume of air that can be inspired over and beyond the normal tidal volume, and it is usually equal to approximately 300ml in the young male adult.
- Expiratory reserve volume is the amount of air that can still be expired by forced expiration after the end of a normal tidal expiration; this normally amounts to about 1100ml in young male adult.
- Residual volume; is the volume of air remaining in the lungs even after the most forceful expiration. This volume averages about 1200ml in young male adult.
- Dead space; this is the amount of air that remains in the tracheal and bronchioles. There is no gaseous exchange in the tube.
- The inspiratory capacity equals the tidal volume plus the inspiratory reserve volume. This is the amount of air (about 3,500ml) that a person can breathe beginning at the normal expiratory level and distending his lungs to the maximum amount
- The functional residual capacity equals the expiratory reserve volume plus the residual volume. This is the amount of air remaining in the lungs at the end of normal expiration (about 2300ml).
- The vital capacity equals the inspiratory reserve volume plus the tidal volume plus the expiratory reserve volume. This is the maximum amount of air that a person can expel in the lungs after first filling his lungs to their maximum extent and then expiring to the maximum extent (about 4,600 ml).
The effect of fluctuation in oxygen and carbon dioxide
A deficient of oxygen (hypoxia) deprives the tissue of the vital requirement for metabolism. The consequence is that the sense organs are impaired, unconsciousness occurs suddenly, followed by paralysis and death.
Breathing pure oxygen at atmospheric pressure present no problems. However, if breathed at pressures greater than atmospheric as in diving, excess oxygen can be dangerous. At first, the tissues metabolise very rapidly, to keep pace with the oxygen supply. As the oxygen build-up, however, it inhibits certain enzymes involved in the Krebs’s cycle, the interfering with respiration.
Cells are more susceptible to changes in the levels of carbon dioxide. An accumulation of this gas increases the acidity of blood and tissue fluids, inhibits enzymes and stops the essential metabolic process. This is why breathing air rich in carbon dioxide is very dangerous.
Control of lung ventilation
In human and other mammals, the overall control of ventilation involves a group of nerve cells comprising a ventilation centre in the posterior part of the brain called the medulla oblongata.
The ‘ventilation centre’ responds to the levels of carbon dioxide and a lesser extent oxygen in the bloodstream.
If the partial pressure of carbon dioxide increases, the centre responds by increasing the ventilation rate and vice versa.
The partial pressure is detected by chemoreceptors found between the internal and external carotid arteries on each side of the neck where they form the carotid bodies and aortic bodies in the walls of the aorta close to the heart.
Gaseous exchange in plants
Plants have numerous stomata (pore) on their leaves and on the green stems or if the stem is woody, through cracks in the bark and via lenticel.
These provide an effective way of gaseous exchange in and out of the plant. H.P Brown and F. Escombe discovered that a greater volume of gas will pass through numerous small holes in a given time than through a single hole of the same total area. This is because diffusion is faster at the perimeter than in the centre of a hole and the combined perimeter of tiny small holes is greater than the perimeter of a few large ones.
The stomata are therefore ideal for gaseous exchange.
Secondly, the spongy mesophyll cell has large air spaces and thus little resistance to the air in the leaves. The opening and closing of stomata can be controlled as the need arises.
Adaptation of animals to low oxygen tension
- High haemoglobin concentration in the blood
- Have myoglobin that store oxygen
- Fish e.g. trout store oxygen in swimming bladder
- Have haemoglobin with high affinity for oxygen e.g. lungworm
- Fish air breath: when oxygen in water is low fishes break the surface and trap air inside the mouth.
- Decreased activities. Animals tend to be sluggish
- Increased ventilation rate i.e. fast and deep breathing to reduce oxygen requirement
- Aquatic surface respiration: fish stay on the surface leave snout at air-water surface taking in water richer in oxygen.
For revision questions and answers download PDF below
Please find free downloadable notes, exams and marking guides of agriculture, biology, Physics, chemistry etc. from digitalteachers.co.ug website.
Dr. Bbosa Science
I need maths notes and chemistry for both O and A level.
go to “digitalteachers.co.ug” under subject tutorial you will find PDF for the required notes
Super-Duper blog! I am loving it! Will come back again. I am taking your feeds also.